SWaP-C Principles Enable Inexpensive High-Definition Thermal Imaging Cameras
Two considerations drive the selection of cameras for volume applications—performance and the burden on resources including limits on accommodating size, weight, power, and cost. For visible-band cameras, both monochrome and RGB, the demands of their highest volume applications—machine vision, surveillance, mobile phones—have driven the performance up and the total resource burden down.
Until recently, no application has placed similar demands on thermal cameras operating in the long-wave infrared (LWIR) band, but now, with the mandate from the U.S.National Highway Traffic Safety Administration (NHTSA) requiring all 2029 cars to include day/night automatic braking, the need for high performance, compact thermal cameras has become urgent.
Thermal imaging has been available for almost 100 years, beginning with a two-pixel camera in 1929, cooled line-scan sensors in the 1960s, cooled area arrays in the 1970s and uncooled microbolometer arrays in the 1980’s. Today, the highest-performance thermal cameras (and most expensive) are cooled but smaller and cheaper uncooled cameras make up the vast majority of units in service.
Even so, the cost of uncooled cameras is tightly tied to performance. The two most important factors are spatial resolution—the number of pixels in the array—and temperature resolution— the smallest difference in temperature in the scene that can be discerned. The variation in requirements for particular applications is substantial.
Demands on Thermal Imaging Cameras Varies By Application
To begin with the most demanding: Critical defense systems demand a spatial resolution of 1024 x 768 or more with a temperature resolution typically less than 50 mK (milliKelvin = 0.001 C). Medical applications may require only modest resolution, perhaps 256 x 320, and thermal resolution below 50 mK for profiling skin temperature. In contrast, architectural thermal leak detection may need only 60 x 80 pixels and thermal resolution of only 1 C.
Related: Biomass One Prevents Wood Pile Fires with Thermal Imaging Solutions
Medical cameras can cost thousands of dollars and handheld thermal leak cameras are just hundreds of dollars. While these prices are certainly lower than the tens of thousands that thermal cameras for defense systems may cost, the upcoming automotive requirements demand far lower costs for next generation thermal cameras that can provide sufficient resolution, both spatial and thermal, to find pedestrians anywhere across a road up to 400 meters away.
Meeting the demanding automotive requirements for cost, performance and tolerance of extreme environments simultaneously will qualify the new camera for use in other volume applications currently served only by visible cameras. Consider:
- Thermal home surveillance system working at night and in rain or fog for longer distance use cases.
- Autonomous vehicles operating safely without natural or artificial light
- Acquisition of thermal data from light, low-cost drones
- Continuous monitoring of production equipment for overheating
- Inspection of new assemblies like circuit boards for hot spots
What exactly do we have to do to reach this goal?
Low-Cost and High-Performance Thermal Cameras
The defense community calls the technique needed SWaP-C. This term is used to focus efforts on reductions in the physical characteristics of Size, Weight, and Power while simultaneously reducing Cost. Successfully achieving this combination requires returning to design principles so that new designs avoid the legacies that would result in increasing cost when the physical parameters are improved.
In uncooled thermal imaging, these legacies include the structure of the microbolometer array, the configuration of the readout circuitry, the methods of implementing image data corrections, and the architecture of the camera electronics. All of these must be reconsidered to achieve the goals of a small, high-performance, low-cost thermal camera.
Taking them in turn, let us start with Size. Cooled cameras are large because the operating temperatures they require necessitate the use of mechanical refrigerators called Stirling cycle coolers. The bulk, reliability, and cost of these drove the change to uncooled cameras. However, uncooled thermal cameras are still large compared to visible cameras with common, practical performance characteristics.
Related: The Beneficial Effects of Thermal Cameras on Emergency Braking Systems
The differences relate to some intractable requirements such as pixel size, which is proportional to the wavelength of the radiation to be detected. An HD (1080p), thermal camera requires a sensor in the range of 2 cm in width while a similar visible sensor can be less than 10% that size. The same factors lead to larger optics for thermal cameras. Size reduction then falls to minimizing the sensor area outside the active array, restricting the package size, and eliminating as much external support circuitry in the camera as possible.
In cameras, the reduction in Weight primarily follows the reduction in size with the additional constraint that the weight of the case and any necessary heat sinks should be minimized and that the connectors chosen themselves should both be light and accommodate lightweight mating connectors and cables. Similarly, the lens mounts and the lenses must be evaluated for weight.
Both size and weight have also long been negatively impacted by the need in uncooled thermal cameras for a mechanical shutter assembly installed in front of the sensor to periodically provide a dark image needed for sensor calibration. Not only are all of the factors in SWaP-C impaired by this shutter but so is the flow of image data, a condition intolerable in systems reliant on continuous data for safety. Eliminating the shutter would offer major improvements in all these factors.
While the shutter is only an intermittent power user, the remainder of the camera is a continuous draw that must be minimized. The primary power users in a thermal camera are the sensor with its readout integrated circuit (ROIC) along with the support circuitry it requires and the drivers to send the signal down the connecting cable. One power reduction opportunity to examine is therefore incorporation of as many external functions as possible into the ROIC design.
Related: Thermal Ranging Technique Delivers Detailed Images
An additional benefit of effectively applying SWaP to the design is that all of these reductions make integration of the camera easier and less costly.
Reducing the Number of Components in Thermal Cameras
Finally, Cost. The most direct way to decrease cost is to reduce the number of components required. Fewer components mean smaller circuit board areas and fewer interconnections between boards. Of course, reducing the number of components only lowers cost when the component prices do not rise uncontrollably so, generally, the components must take advantage of standard manufacturing processes already applied to other low-cost products. External cost impacts must also be considered—optics, cabling, power supplies, and protection.
Fortunately, the suite of SWaP-C improvement techniques can be applied to the design of a thermal camera, assuming that the designer is willing to set aside many of the traditional camera design choices. Specifically, an entire set of improvements is enabled if the sensor is redesigned to provide digital data rather than the traditional analog video signal.
Designing Digital Thermal Sensors
Beginning with the obvious: If the sensor has a digital output, then external conversion is not needed either inside the camera or as part of an image acquisition system. This allows use of one of many standard digital interfaces that are natively supported in computers. The camera becomes just another peripheral.
Making the ROIC digital enables some of the external digital processing functions to be moved on-chip. Most importantly, a suitable analog-to-digital conversion architecture could accommodate corrections for sensor non-uniformity, both offset and gain, as part of the digitizing process. The data used for these corrections could be a combination of patterns developed during sensor tests and real-time measurements of sensor characteristics such as temperature. Then, the corrections could be applied using memory and computational capabilities within the ROIC itself. Such a sensor needs no mechanical shutter and so can correct itself without ever interrupting the image data flow.
Related: 11 Myths About Thermal Imaging for Cars
One significant additional benefit of providing the ROIC with digital functionality is the possibility of including protection against use of the thermal camera in restricted applications. For instance, a surviving camera in a crashed drone could be prevented from operating in equipment used by an adversary if it were automatically disabled whenever loss of the authorized host was detected. It is precisely this capability, most securely implemented inside the ROIC, that can allow high-performance thermal cameras to be shipped globally in automobiles without trade licensing.
Standard device fabrication processes can produce such an ROIC. What remains then is to use another set of existing processes to build the microbolometer array on the ROIC and then, in a wafer-scale operation, provide the vacuum environment the microbolometers need for accurate thermal detection. Figure 2 shows the result with a comparison to the structure of a traditional microbolometer array with support circuitry. Note especially that the array covers the entire ROIC, eliminating wasted area and improving the resolution from VGA to 1280 x 800.
With the array covering the entire ROIC, the package for the device can be only slightly larger than the device itself when it is mounted in a circuit board in a way that permits rear heatsinking. The board area needed is also not much larger than the active sensor area. Because all of the necessary data conditioning is performed on the ROIC, only power conditioning and data drivers are needed on the board. The result is illustrated in Figure 3, which shows a complete camera on a single board ready to accept a single power supply and to transmit real-time corrected video data over a high-speed digital link.
Related: Spinning Meta-Optics for Spectro-Polarimetric Thermal Imaging?
To the camera module, the user has only to add an enclosure with a small rear heat sink and a mounting fixture for a lens. Both power and digital video are carried by a single cable.
This high-definition camera design attains the goal of the SWaP-C philosophy: It is small and light, consuming minimal power and requiring only a simple enclosure and a single external connection. Cost is constrained through the use of standard manufacturing processes and by the elimination of any need for complex auxiliary electronics.
High performance, low cost, and low resource burden make this design ideal for many high-volume thermal imaging applications.
About the Author
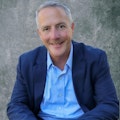
Wade Appelman
Wade Appleman, chief business officer at Owl Autonomous Imaging, Owl AI, (Fairport, NY, USA), has held leadership roles in both private and public sector organizations. Appleman has led sales and marketing efforts for five venture-backed startups with toal exit valuations of $900 million. He also helped launch to market several companies including SensL Technologies, which was acquired by On Semiconductor (now onsemi) in May 2018. SensL was a leader in single photon detection used in LiDAR and autuomation.
He also held leadership positions at onsemi (Phoenix, AZ, USA) after the acquisition, Vitesse Semiconductor (Camarillo, CA, USA), and Cabletron Systems, which ceased operations in 2001.